This page contains the complete chapter.
1
Matter, Space and Time
Before introducing the new concept of wisp theory, we begin by briefly
by reviewing our current understanding of what matter, space and time
are, while making comparisons with wisp theory along the way.
1.1 Basic understanding
1.1.1 Matter
The ancient Greek philosophers Leucippus and Democritus thought that matter
was made from small indivisible lumps called atoms (the Greek atomos means
uncuttable).
We can tell the shape of matter from the light coming off its surface.
We can feel it by touch through interaction with its electromagnetic
force repelling our attempts to compress it. And we can calculate its
mass its reluctance to accelerate when force is applied.
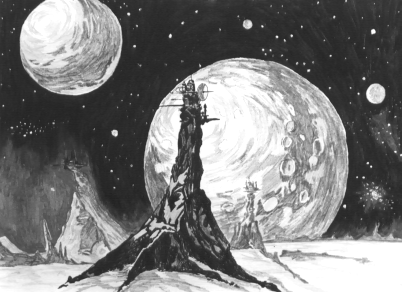
Figure 1.1 Scene showing matter - heavenly bodies - in
space
Figure 1.1 shows a space scene: large planet-sized lumps
of matter moving through the void. Here our senses guide our thoughts
to consider matter as being something hard and solid.
1.1.2 Space
It is a three-dimensional volume that can be filled with something or
can be empty. We believe that most of space is empty and that matter can
move through it effortlessly.
1.1.3 Time
It is a dimension that enables two otherwise identical events that occur
at the same point in space to be distinguished.
Isaac Newton thought that time was the measure of an absolute quantity
that is the same throughout the universe and independent of an observers
position or speed.
1.1.4 Perception of reality
Our perception of reality is strongly determined by our visual sense.
It is easy to see how early models of atoms were compared to a miniature
model of the solar system. Even today the notion that subatomic particles
are tiny points of matter is taken very seriously.
You will soon discover that the opposite of what our senses perceive is
in fact the reality matter is empty and space is full.
1.2 Advanced understanding
1.2.1 Matter
1.2.1.1 Quantum theories
In 1900 Max Planck devised quantum theory to account for the emission
of black-body radiation from hot bodies. He observed that radiation is
emitted in discrete packets, or quanta of energy.
In the 1920s advances in this theory led to the development of quantum
mechanics, in which matter is described as being both particles with mass
and energy, and wave packets wobbles with mass and energy. But
quantum mechanics is only a mathematical tool used to describe the behaviour
of matter with its dual wave-particle property, it does not actually
tell us what matter is! Good advice to physicists studying quantum mechanics
is Dont waste time trying to understand how it works, just
use it to calculate results. It works.
Erwin Schrödinger an early pioneer of quantum theory and discoverer
of the quantum mechanics wave equation of a particle (1927) admitted
that he did not really understand why matter behaved this way.
1.2.1.2 Fields
Many physicists believe that the fundamental material entities are fields,
where particles are formed by disturbances in the fields. In quantum field
theory first proposed by Paul Dirac in 1927 particles are
represented by quantized oscillations in the fields. Wisp theory supports
this view, but suggests that the disturbances that form particles are
primarily geometric in nature fractals and wave oscillations
are a secondary feature. Also, wisp space comprises discrete-sized particles,
and so it does not form a continuous field medium.
1.2.1.3 Mass
The legendary Richard Feynman wrote in his book: QED, The Strange Theory
of Light and Matter:
- Throughout this entire story there remains one especially unsatisfactory
feature: the observed masses of the particles, m. There is no theory
that adequately explains these numbers. We use the numbers in all our
theories, but we dont understand them what they are, or
where they come from. I believe that from a fundamental point of view,
this is a very interesting and serious problem.
1.2.1.4 Mass energy equivalence
Albert Einstein has shown that mass and energy are two forms of the same
thing, which are related by the equation E = mc^2. This important relationship
will be explored in detail in a later chapter. Matter is also subject
to relativistic effects at very high speeds its mass appears to
increase the faster it moves.
Even though Einstein has shown that mass and energy are interchangeable,
do we really understand the process involved?
Wisp theory will enable you to visualise the massenergy interaction.
1.2.1.5 The standard model
Physicists have developed the standard model (Figure 1.2) and are constantly
testing new discoveries against it. They have verified the existence of
many point-like fundamental particles and are currently searching for
the elusive Higgs boson, believed to be the fundamental particle that
gives matter its mass; if found, it will add support to the standard model.
Scientists at CERN the European Laboratory for Particle Physics
near Geneva in Switzerland continue their search. However, it looks
increasingly unlikely that it will show up, raising doubt about the standard
model.
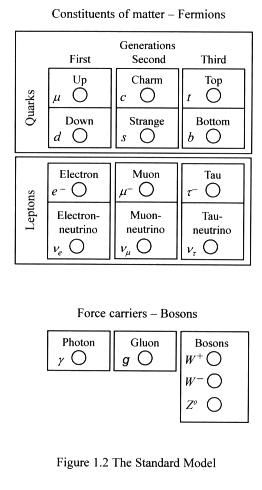
1.2.1.6 Supersymmetry theory
A quarter of a century ago, Julius Wess and Bruno Zimino proposed supersymmetry
theory that has to do with quantum-mechanical spin. When the ideas of
supersymmetry were applied to the standard model, it suggested the existence
of new elementary particles that allow bosons and fermions to form particle
pairs. Every boson has a corresponding fermion partner and every fermion
has a corresponding boson partner.
So far the new particle pairs predicted have not been detected.
1.2.1.7 String theories
Michael Green and John Schwarz continued development of string theory
discovered in 1968 by Gabriele Veneziano and improved on in 1970
by Yoichiro Nambu, Holger Nielsen and Leonard Susskind and in 1984
they released superstring theory. It suggests that matter is made from
incredibly small one-dimensional quantum strings 10^-35 m in length that
exist in a 10-dimensional environment six hidden and four visible
to us.
These strings have no mass like light; they spin, vibrate and rotate,
yielding different quantum energy states. Their energy states or harmonics
correspond to different fundamental particles within the same family.
The extra invisible dimensions can be regarded as mathematical artefacts.
David Gross later added 16 extra dimensions to account for bosons
the transmitters of force. A total of 10 dimensions are needed for fermions,
and 26 dimensions are needed for bosons in order to be consistent with
quantum theory.
Superstring theory (string theory for short) has incorporated supersymmetry
in an attempt to unify the four fundamental forces of nature. But physicists
are still a long way from being able to say whether string theory is correct.
1.2.1.8 M-Theory
Since the mid-1990s, Edward Witten has been developing M-theory (membrane
theory) from string theory. It focuses on the symmetry links between equations
and adds an extra 11th dimension to support gravity.
String theory and M-theory have so far not achieving their main goal in
becoming the theory of everything.
1.2.2 Space and time
1.2.2.1 Einsteins spacetime
Einsteins spacetime is a relative quantity. Observers in motion
with respect to one another will measure their spacetime components
differently; they will age at different rates; and record different times
for similar events.
The notion that space and time are joined together is now universally
accepted. Einsteins relativity theories the special theory,
proposed in 1905, and general theory, proposed in 1915 were developed
around this concept. Although it is counter-intuitive that space and time
should be joined; Einsteins theories are strongly supported by experiment.
However, it is interesting to note that Hendrik Lorentz whose formula
is central to Einsteins special theory of relativity was
critical of the spacetime link. Why? Because the lose of simultaneity
for separated events defies common sense. Also it should be noted that
quantum theory does not require that space and time be joined.
1.2.2.2 String theorys spacetime
In string theory, the vibrating, rotating, one-dimensional string essentially
creates spacetime. Remove the string and spacetime would cease
to exist.
1.2.2.3 Time dilation
Einstein predicted the effect of time dilation from his special theory
of relativity the flow of time slows for bodies in motion. And
it is an established fact, that muons (created in the Earths upper
atmosphere by high-energy cosmic rays striking oxygen and nitrogen nuclei),
moving at near light-speed, age more slowly than those travelling at slower
speeds do.
The effect of time dilation has been proven correct many times over and
is supported by wisp theory.
1.2.2.4 Unit of time
In 1967 a natural unit of time was adopted (SI units), based upon the
caesium atom (atomic clock). One second is defined as the time required
for a caesium atom to vibrate exactly 9,192,631,770 times.
1.2.2.5 Demise of the ether
Space was at one time thought to consist of ether, a hypothetical substance
that filled all of space and was responsible for the propagation of electromagnetic
waves such as light. However, the famous null result
of an experiment measuring the Earths speed through the supposed
ether carried out by MichelsonMorley in 1887, gave scientists
good reason to doubt its existence. And finally, when Einstein published
his special theory of relativity in 1905, the fate of the ether was sealed.
1.3 Incompatible theories
The two great theories of the twentieth century: general relativity and
quantum mechanics are totally incompatible and cannot be unified. The
difficulty in merging these is due to the spacetime link. Whereas
quantum mechanics treat space and time as being separate, general relativity
does not. If general relativity is flawed because of this link, then string
theories likewise are flawed.
String theorists attempted unification by adding an extra dimension to
account for gravity. This creates quantum gravity whose force carrier
is predicted to be the graviton. But so far the graviton has not been
detected, and so unification is incomplete.
Also they had considered building their theories using rotating, vibrating,
three-dimensional blobs. But encountered problems with relativistic covariance
because relativistic equations join space and time, and so the
objects they used could only be one-dimensional. Once the link is broken,
they will have the freedom to revise their theories.
Wisp theory builds an ether relativity theory, which treat
space and time as being separate, breaking the link, and making unification
possible.
1.4 Theory foundations roots
The long-term success of any theory relies to a large extent on the strength
of its founding principles. It is important when dealing with complex
problems to be able to work back to the roots of a theory. By doing so,
you can check that the theory remains valid and has a practical basis.
Newtons theory of gravity and his laws of motion are simple and
easy to understand. From Newtons equations we are able to calculate,
for example, how galaxies move, and to plan space missions to the planets
in the solar system. The successes of his theories are based upon his
ability to use powerful analytical skills to simplify complex problems.
He developed his theories hand-in-hand with experimental observation,
constantly cross-checking his work.
Quantum theory also developed using powerful logical reasoning coupled
to strange experimental observations particles behaving as waves
and vice versa. It too has been built upon solid foundation that should
ensure longevity. It uses mathematical tools of complex artefact nature.
But nevertheless, theory predictions appear correct and have been verified
to very high degrees of accuracy.
Einsteins special relativity is also based on powerful reasoning
and simple structure. It appears to be supported by all experimental observations,
so that it should have longevity. But there are many aspects of the theory
that have to be taken on trust, simply because we do not have the technology
to test it fully. And some aspects of the theory defy common sense: the
joining of space and time, and the breakdown of simultaneity of events.
There is no denying that Einsteins relativity theories are powerful,
carefully constructed, and based on clear founding principles. But with
any theory, we should always question its truth, constantly probing it
for signs of weakness.
String theories are highly abstract in nature. They deal in spacetime
dimensions that are beyond the reach of experimental observation. They
use highly complex abstract mathematical tools to build models that may
not even exist in the real world. But their results may reveal new insights
into how the universe works. However, the weakness with such an approach
is that it has no solid foundation and lacks clear direction. Its predictions
cannot be traced back to its roots.
1.4.1 Wisp theory roots/history
Wisp theory develops around one simple principle discovered by
chance in the local town library on 11th December 1993.
Prior to that powerful thought, many years earlier I had concluded that
matter should not be able to interact. For example, if the smallest possible
piece of matter is made of a hard substance surrounded by empty space,
then there is no conceivable way in which a force could cause two such
pieces to move together or push apart. Consider a line of force between
two pieces of matter. There is no physical means possible by which a line
can cause them to pull together or push apart.
If on the other hand the transfer of force is caused by a force particle,
say the graviton, then again the physical process by which the pieces
pull together is impossible. How can a graviton a particle
moving between two pieces of matter, cause them to move together!
Following the above reasoning, particles of matter cannot interact by
such means. They should in fact drift aimlessly about, occasionally bumping
into one another. The universe should in fact be dead!
These were thoughts from my early years. Such thoughts were not academically
constructive and consequently I did not pursue a career in physics.
In 1994 I drafted the first version of wisp theory. My plan was to keep
it simple and document all details so they could be carefully checked.
The first draft included a new theory of gravity that not only agreed
with Newtons law of gravitation, but also in principle supported
his idea as to its cause density variation in space.
I developed a relativity theory based on wisp theorys principles.
Tests run on a computer showed that wisp relativity and special relativity
did not agree. Although I was confident that wisp relativity was correct
and special relativity was wrong, how could I challenge Einstein with
such a simple theory?
In 1995 I started a degree course in science with the Open University.
After completing my degree I was made redundant company relocation
and took the opportunity to complete work on wisp theory during
2002.
This book is a complete rewrite of the original draft and a much-improved
theory. But any theory that challenges special relativity will be subject
to severe criticism. Whether the theory gets established will depend on
support from theoretical and experimental physicists who have the knowledge
and skills to test it properly. I believe it is correct and that it will
enable scientists to make many new discoveries.
|